The tagline of the CGIAR Generation Challenge Programme (GCP) is ‘Partnerships in modern crop breeding for food security’. One of GCP’s many rewarding partnerships was with the International Crops Research Institute for the Semi-Arid Tropics (ICRISAT).
The Institute was a source of valuable partnerships with highly regarded agricultural scientists and researchers, as well as of germplasm and genetic resources from its gene bank. With assistance from GCP, these resources have enabled scientists and crop breeders throughout Africa, Asia and Latin America to achieve crop improvements for chickpea, groundnut, pearl millet, pigeonpea and sorghum, all of which are staple crops that millions of people depend upon for survival.
“The philosophy of GCP at the start was to tap into and use the genomic recourses we had in our gene banks to develop ICRISAT’s and our partners’ breeding programmes,” says Pooran Gaur, GCP’s Product Delivery Coordinator for chickpeas, and Principal Scientist for chickpea genetics and breeding at ICRISAT.
ICRISAT’s gene bank is a global repository of crop genetic diversity. It contains 123,023 germplasm accessions – in the form of seed samples – assembled from 144 countries, making it one of the largest gene banks in the world.
The collection serves as insurance against genetic loss and as a source of resistance to diseases and pests, tolerance to climatic and other environmental stresses, and improved quality and yield traits for crop breeding.
Pooran says the ultimate goal of the GCP–ICRISAT partnership was to use the resources in the gene bank to develop drought-tolerant varieties that would thrive in semi-arid conditions and to make these varieties available to farmers’ fields within a decade.
Setting a foundation for higher yielding, drought-tolerant chickpeas
Pooran was involved with GCP from its beginning in 2004 and was instrumental in coordinating chickpea projects.
“GCP got things started; it set a foundation for using genomic resources to breed chickpeas,” says Pooran. During Phase I of GCP (2004–2009), ICRISAT was involved in developing reference sets for chickpeas and developing mapping populations for drought-tolerance traits. It also collaborated with 19 other international research organisations to successfully sequence the chickpea genome in 2013 – a major breakthrough that paved the way for the development of even more superior chickpea varieties to transform production in semi-arid environments.
The International Chickpea Genome Sequencing Consortium, led by ICRISAT and partly funded by GCP, identified more than 28,000 genes and several million genetic markers. Pooran says these are expected to illuminate important genetic traits that may enhance new varieties.
The trait of most interest to many chickpea breeders is drought tolerance. In recent years, droughts in the south of India, the largest producer of chickpeas, have reduced yields to less than one tonne per hectare. Droughts have also diminished chickpea yields in Ethiopia and Kenya, Africa’s largest chickpea producers and exporters to India. While total global production of chickpeas is around 8.6 million tonnes per year, drought causes losses of around 3.7 million tonnes worldwide.
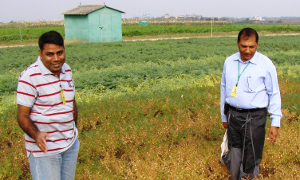
Putting it to the test: Rajeev Varshney (left, see below) and Pooran Gaur (right) inspecting a chickpea field trial.
Pooran says the foundation work supported by GCP was particularly important for identifying drought-tolerance traits. “We had identified plants with early maturing traits. This allowed us to develop chickpea varieties that have more chance of escaping drought when cereal farmers produce a fast-growing crop in between the harvest and planting of their main crops,” he says.
New varieties that grow and develop more quickly are expected to play a key role in expanding the area suitable for chickpeas into new niches where the available crop-growing seasons are shorter.
“In southern India now we are already seeing these varieties growing well, and their yield is very high,” says Pooran. “In fact, productivity has increased in the south by about seven to eight times in the last 10–12 years.”
Developing capacity by involving partners in Kenya and Ethiopia
As part of GCP’s Tropical Legumes I project (TLI), incorporated within its Legume Research Initiative (RI), ICRISAT partnered with Egerton University in Kenya and the Ethiopian Institute of Agricultural Research (EIAR) to share breeding skills and resources to produce higher yielding, drought-tolerant chickpea varieties.
“When we first started working on this project in mid-2007, our breeding programme was very weak,” says Paul Kimurto of the Faculty of Agriculture at Egerton University, who was Lead Scientist for chickpea research in the TLI project. “We have since accumulated a lot of germplasm, a chickpea reference set and a mapping population, all of which have greatly boosted our breeding programme.”
Paul says that with this increased capacity, his team in Kenya had released six new varieties of chickpea in the five years prior to GCP’s close at the end of 2014, and were expecting more to be ready within in the next three years.
In fields across Ethiopia, meanwhile, the introduction of new varieties has already brought a dramatic increase in productivity, with yields doubling in recent years, according to Asnake Fikre, Crop Research Directorate Director for EIAR.
Varieties like the large-seeded and high-valued kabuli have presented new opportunities for farmers to earn extra income through the export industry, and indeed chickpea exports from eastern Africa have substantially increased since 2001. This has transformed Ethiopia’s chickpeas from simple subsistence crop to one of great commercial significance.
Decoding pigeonpea genome
Two years prior to the decoding of the chickpea genome, GCP’s Director Jean-Marcel Ribaut announced that a six-year, GCP-funded collaboration led by ICRISAT had already sequenced almost three-quarters of the pigeonpea genome.
“This will have significant impact on resource-poor communities in the semi-arid regions, because they will have the opportunity to improve their livelihoods and increase food availability,” Jean-Marcel stated in January 2012.
Pigeonpea, the grains of which make a highly nutritious and protein-rich food, is a hardy and drought-tolerant crop. It is grown in the semi-arid tropics and subtropics of Asia, Africa, the Americas and the Caribbean. This crop’s prolific seed production and tolerance to drought help reduce farmers’ vulnerability to potential food shortages during dry periods.
The collaborative project brought together 12 participating institutes operating under the umbrella of the International Initiative for Pigeonpea Genomics. The initiative was led by Rajeev K Varshney, GCP’s Genomics Theme Leader and Director of the Center of Excellence in Genomics at ICRISAT. Other participants included BGI in Shenzhen, China; four universities; and five other advanced research entities, both private and public. The Plant Genome Research Program of the National Science Foundation, USA, also funded part of this research.
“We were able to assemble over 70 percent of the genome. This was sufficient to enable us to change breeding approaches for pigeonpea,” says Rajeev. “That is, we can now combine conventional and molecular breeding methods – something we couldn’t do as well before – and access enough genes to create many new pigeonpea varieties that will effectively help improve the food security and livelihoods of resource-poor communities.”
Pigeonpea breeders are now able to use markers for genetic mapping and trait identification, marker-assisted selection, marker-assisted recurrent selection and genomic selection. These techniques, Rajeev says, “considerably cut breeding time by doing away with several cropping cycles. This means new varieties reach dryland areas of Africa and Asia more quickly, thus improving and increasing the sustainability of food production systems in these regions.”
Several genes, unique to pigeonpea, were also identified for drought tolerance by the project. Future research may find ways of transferring these genes to other legumes in the same family – such as soybean, cowpea and common bean – helping these crops also become more drought tolerant. This would be a significant asset in view of the increasingly drier climates in these crops’ production areas.
“We cannot help but agree with William Dar, Director General of ICRISAT, who observed that the ‘mapping of the pigeonpea genome is a breakthrough that could not have come at a better time’,” says Jean-Marcel.
Securing income-generating groundnut crops in Africa
Groundnut, otherwise known as peanut, is one of ICRISAT’s mandate crops. Groundnuts provide a key source of nutrition for Africa’s farming families and have the potential to sustain a strong African export industry in future.
“The groundnut is one of the most important income-generating crops for my country and other countries in East Africa,” says Patrick Okori, who is a groundnut breeder and Principal Scientist with ICRISAT in Malawi and who was GCP’s Product Delivery Coordinator for groundnuts.
“It’s like a small bank for many smallholder farmers, one that can be easily converted into cash, fetching the highest prices,” he says
It is the same in West Africa, according to groundnut breeder Issa Faye from the Institut Sénégalais de Recherches Agricoles (ISRA), who has been involved in GCP since 2008. “It’s very important for Senegal,” he says. “It’s the most important cash crop here – a big source of revenue for farmers around the country. Senegal is one of the largest exporters of groundnut in West Africa.”
In April 2014, the genomes of the groundnut’s two wild ancestral parents were successfully sequenced by the International Peanut Genome Initiative – a multinational group of crop geneticists, including those from ICRISAT, who had been working in collaboration for several years.
The sequencing work has given breeders access to 96 percent of all groundnut genes and provided the molecular map needed to breed drought-tolerant and disease-resistant higher yielding varieties, faster.
“The wild relatives of a number of crops contain genetic stocks that hold the most promise to overcome drought and disease,” says Vincent Vadez, ICRISAT Principal Scientist and groundnut research leader for GCP’s Legumes Research Initiative. And for groundnut, these stocks have already had a major impact in generating the genetic tools that are key to making more rapid and efficient progress in crop science
Chair of GCP’s Consortium Committee, David Hoisington – formerly ICRISAT’s Director of Research and now Senior Research Scientist and Program Director at the University of Georgia – says the sequencing could be a huge step forward for boosting agriculture in developing countries.
“Researchers and plant breeders now have much better tools available to breed more productive and more resilient groundnut varieties, with improved yields and better nutrition,” he says.
These resilient varieties should be available to farmers across Africa within a few years.
Supporting key crops in West Africa
With a focus on the semi-arid tropics, ICRISAT has been working closely with partners for 30 years to improve rainfed farming systems in West Africa. One sorghum researcher who has been working on the ground with local partners in Mali since 1998 is Eva Weltzien-Rattunde. She is an ICRISAT Principal Scientist in sorghum breeding and genetic resources, based in Mali, and was Principal Investigator for GCP’s Sorghum Research Initiative.
Eva and her team collaborated with local researchers at Mali’s Institut d’Economie Rurale (IER) and France’s Centre de coopération internationale en recherche agronomique pour le développement (CIRAD; Agricultural Research for Development) on a project to test a novel molecular-breeding approach: backcross nested association mapping (BCNAM). Eva says this method has the potential to halve the time it takes to develop local sorghum varieties with improved yield and adaptability to poor soil fertility conditions.
In another project, under GCP’s Comparative Genomics Research Initiative, Eva and her team are using molecular markers developed through the RI to select for aluminium-tolerant and phosphorus-efficient varieties and validating their performance in field trials across 29 environments in three countries in West Africa.
“Low phosphorus availability is a key problem for farmers on the coast of West Africa, and breeding phosphorus-efficient crops to cope with these conditions has been a main objective of ICRISAT in West Africa for some time,” says Eva.
“We’ve had good results in terms of field trials. We have at least 20 lines we are field testing at the moment, which we selected from 1,100 lines that we tested under high and low phosphorous conditions.” Eva says that some of these lines could be released as new varieties as early as 2015.
Ibrahima Sissoko, a data curator working with Eva’s team at ICRISAT in Mali, also adds that the collaborations and involvement with GCP have increased his and other developing country partners’ capacity in data management and statistical analysis, as well as helping to expand their network. “I can get help from other members of my sorghum community,” he says.
In summing up, Eva says: “Overall, we feel the GCP partnerships are enhancing our capacity here in Mali, and that we are closer to delivering more robust sorghum varieties that will help farmers and feed the ever-growing population in West Africa.”
Tom Hash, millet breeder and Principal Scientist at ICRISAT and GCP Principal Investigator for millet, shares Eva’s sentiments on GCP and the impact it is having in West Africa.
Between 2005 and 2007, GCP invested in genetic research for millet, which is the sixth most important cereal crop globally and a staple food (along with sorghum) in Burkina Faso, Chad, Eritrea, Mali, Niger, northern Nigeria, Senegal and Sudan.
With financial support from GCP, and drawing on lessons learnt from parallel GCP genetic research, including in sorghum and chickpea, ICRISAT was able to mine its considerable pearl millet genetic resources for desirable traits.
Hari D Upadhyaya, Principal Scientist and Head of Genebank at ICRISAT in India, led this task to develop and genotype a ‘composite collection’ of pearl millet. The team created a selection that strategically reduced the 21,594 accessions in the gene bank down to just 1,021. This collection includes lines developed at ICRISAT and material from other sources, with a range of valuable traits including tolerance to drought, heat and soil salinity and resistance to blast, downy mildew, ergot, rust and smut, and even resistance to multiple diseases.
The team then used molecular markers to fingerprint the DNA of plants grown from the collection.
“GCP supported collaboration with CIRAD, and our pearl millet breeding teams learnt how to do marker-based genetic diversity analysis,” says Tom. “This work, combined with the genomic resources work, did make some significant contributions to pearl millet research.”
Over 100 new varieties of pearl millet have recently been developed and released in Africa by the African Centre for Crop Improvement in South Africa, another developing country partner of ICRISAT and GCP. Tom says the initial genetic research was pivotal to this happening.
From poverty to prosperity through partnerships
Patrick Okori says that GCP has enabled his organisation to make a much stronger contribution to the quality of science.
“Prior to GCP, ICRISAT was already one of the big investors in legume research, because that was its mandate. The arrival of GCP, however, expanded the number of partners that ICRISAT had, both locally and globally, through the resources, strategic meetings and partnership arrangements that GCP provided as a broad platform for engaging in genomic research and the life sciences.”
This expansion of ICRISAT, facilitated by GCP, also enabled researchers from across the world and in diverse fields to interact in ways they had never had the opportunity to before, says Vincent Vadez.
“GCP has allowed me to make contact with people working on other legumes, for example,” he says. “It has allowed us to test hypotheses in other related crops, and we’ve generated quite a bit of good science from that.”
Pooran Gaur has had a similar experience with his chickpea research at ICRISAT.
“GCP provided the first opportunity for us to work with the bean and cowpea groups, learning from each other. That cross-learning from other crops really helped us. You learn many things working together, and I think we have developed a good relationship, a good community for legumes now.”
This community environment has made the best use of an unusual variety of skills, knowledge and resources, agrees Rajeev Varshney.
“It brought together people from all kinds of scientific disciplines – from genomics, bioinformatics, biology, molecular biology and so on,” he says. “Such a pooling of complementary expertise and resources made great achievements possible.”
More links
- Here on the Sunset Blog: chickpea | groundnut | pearl millet | sorghum | comparative genomics
- Blogposts on the GCP blog: chickpea | groundnut | sorghum | comparative genomics
- Research Initiatives: legumes | comparative genomics